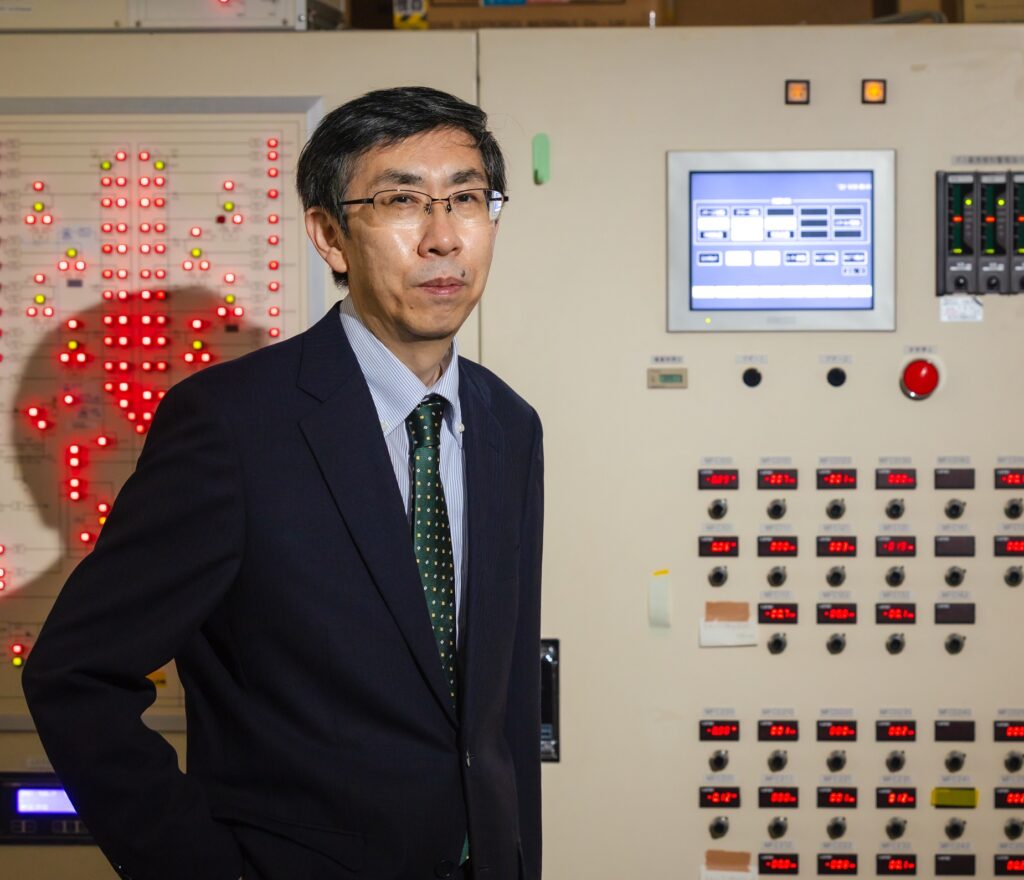
Professor Shimomura Kazuhiko of the Faculty of Science and Technology researches and develops new optical communication devices integrated with computer LSI. He outlines why such research is needed right now, and also talks about bonding technology for silicon platform that is being developed for practical applications.
Smartphones and computers are an essential part of our lives today—the volume of data accessed via the internet from devices such as these has increased by an average of 30% annually compared to ten years ago. A sudden increase of 57.4% in 2020 compared to the previous year can be attributed to the rise in people working from home following the outbreak of COVID-19. Being able to access the internet without any major issues under such conditions is due to an increase in optical communications capable of sending vast quantities of data at high speeds. Yet lingering technical issues mean some sections still operate electrically instead of using optical communications.
One example of this is data centers that operate as relay facilities for connecting to the internet. These centers operate 24-hours a day and house hundreds of thousands of computer servers that are available for users, but the integrated circuits between those servers and inside the computers are based on electrical wiring. The amount of power used by Google’s data centers in the US is said to be around that generated by one whole nuclear power plant, and the key issue is just how to secure such a huge amount of power. If new optical communication devices can be developed, electrical energy can be used more efficiently as the use of optical communications increases.
Any gap in joints prevent conversion to light
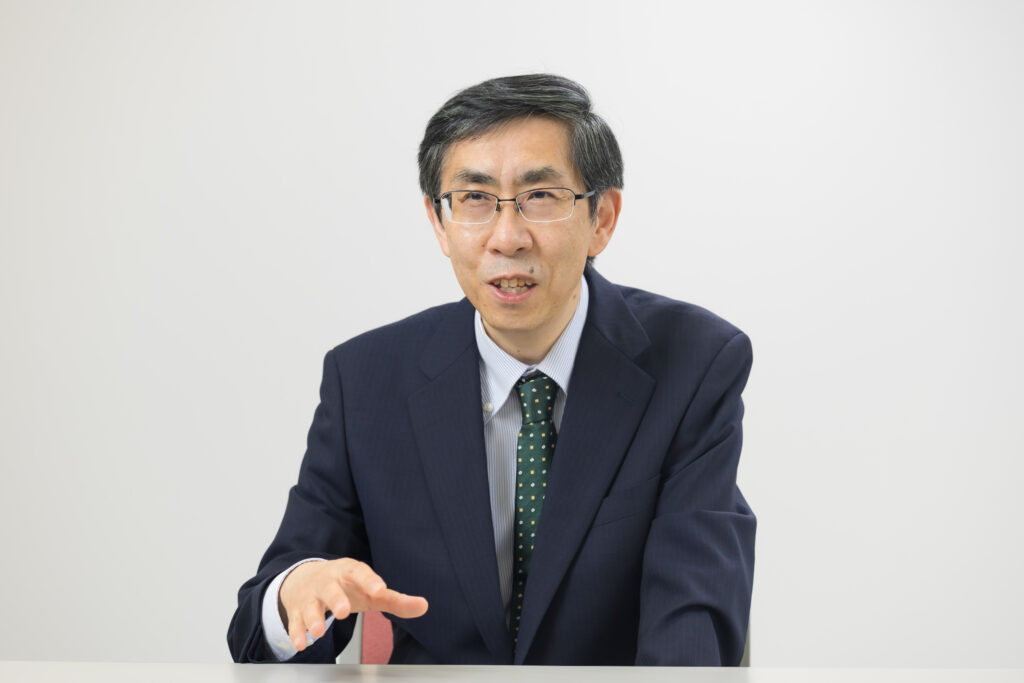
The optical devices that I focus on are “silicon-based lasers” mounted to LSI circuits of computers—I am trying to develop devices that operate using photonics instead of the electrical wiring that has been common until now. To achieve this, we need to develop a way that converts electrical signals sent through LSI into flashing lights using semiconductor lasers. Yet LSI substrates are made of silicon, which does not work well with light—signals failed to be converted to light even when bonded with indium phosphide, a semiconductor used as the laser light source.
As we advanced our research, we found that any air bubbles or gaps in the bonds between the silicon and indium phosphide prevents light from being transmitted. To overcome this, we came up with the method of adding a one-micron thick layer of indium phosphide—thinner than gold leaf. This method was successful in emitting light.
Achieved a breakthrough following advice from colleagues
The next challenge we faced was with the durability of the layer emitting light. This layer ends up degrading with heat when current is passed through it, so we needed to constantly cool the substrate down. This adversely affected potential practical applications, so we studied different methods capable of increasing the strength of the layer—in 2016 we succeeded in converting electricity to light at room temperature. Yet this was achieved by using pulsed currents, where the current is changed into instantaneous pulses, so we are continuing our research efforts with the aim of being able to emit light continuously using direct current.
We faced a dead-end in the year or two after we began our research, after producing thousands of chips that eventually failed. What helped us get through this difficult time was our fellow researchers at academic societies and other universities—the advice we received from other researchers into semiconductor lasers in particular helped us to achieve the breakthrough we needed. The motivating force of my research comes from being able to work with fellow researchers, and the desire to give back to society through my research efforts. I hope to develop practical applications of this technology so that it can play a role in alleviating energy-related problems as soon as possible.
More recently, I have also been involved in research into semiconductor nanowire structures. A nanowire is a form of wire in the nanometer scale, or one-millionth of a millimeter. Arranging semiconductors into a nanowire layout increases its surface area, which may have practical uses in applications like solar cells. This research is related to energy-efficiency like optical devices, and is a very rewarding field of study.
The book I recommend
“Jiryoku to juryoku no hakken Volume 3: Kindai no hajimari”(The Pull of History: The Dawn of the Modern Age)
by Yoshitaka Yamamoto, Misuzu Shobo
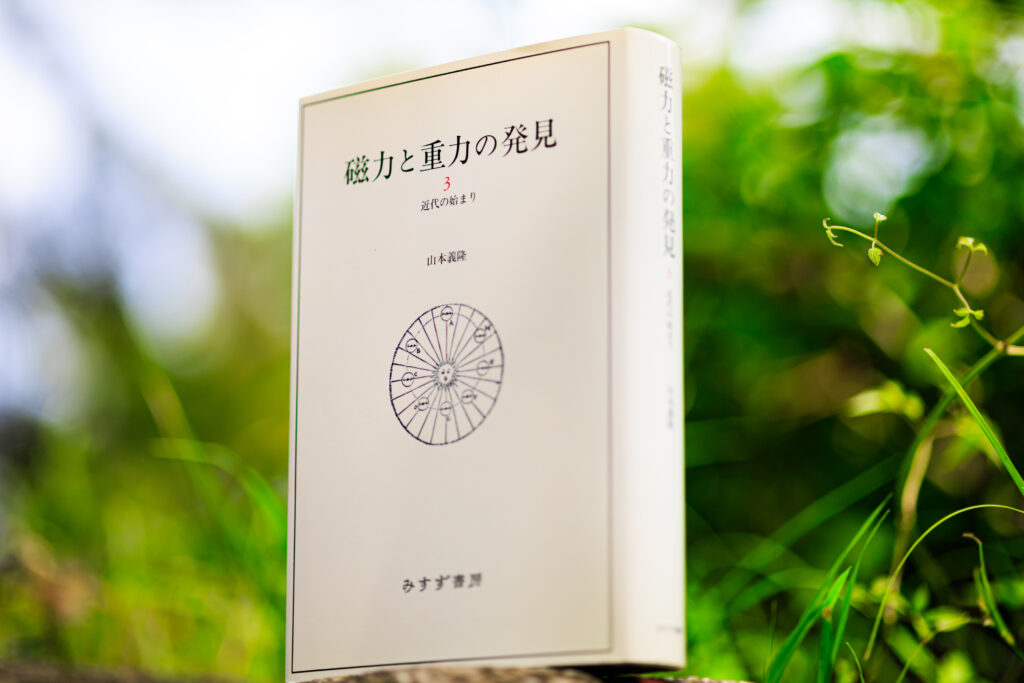
The third volume focusing on the modern age, in a series covering Antiquity and the Middle Ages in Volume 1, and the Renaissance in Volume 2. Reading the entire series provides a valuable insight into the history of electromagnetism. This is all presented as a story, from electromagnetism being viewed as a form of black magic in the Middle Ages, to the rapid development of modern physics in the wake of experiments conducted by physicist Coulomb.
-
Kazuhiko Shimomura
- Professor
Department of Engineering and Applied Sciences
Faculty of Science and Technology
- Professor
-
Graduated with a degree in Physical Electronics from the School of Engineering of the Tokyo Institute of Technology, and received his M.S. and Ph.D in engineering from the university’s Graduate School of Science and Engineering. Worked as a research associate at the Tokyo Institute of Technology, full-time lecturer and assistant professor at the Faculty of Science and Technology of Sophia University, and his current position from 2002.
- Department of Engineering and Applied Sciences
Interviewed: June 2022